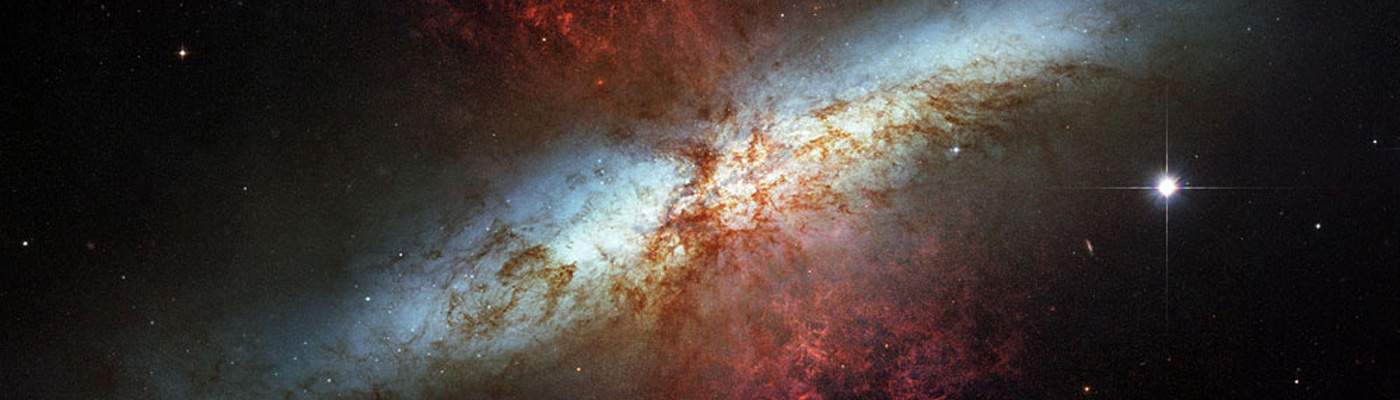
Cosmology
Cosmology is the study of the Universe as a whole rather than individual objects.
Our researchers
- Richard Battye
- Michael Brown (Theme lead)
- Ian Browne
- Jens Chluba
- Chris Conselice
- Clive Dickinson
- Keith Grainge
- Neal Jackson
- Scott Kay
- Lucio Piccirillo
- Ralph Spencer
- Peter Wilkinson
- Laura Wolz
It involved the study of the origins and ultimate fate of the Universe constrained using observations of the Cosmic Microwave Background and Large-Scale Structure.
JBCA cosmology is playing significant roles in a number of areas and is involved in many high profile observational projects such as Planck, Euclid, Dark Energy Survey (DES), Large Synoptic Survey Telescope (LSST) and the Square Kilometre Array (SKA).
Key questions which we are interested in are:
- the understanding the origins of the initial deviations for homogeneity that eventually lead to the formation of large scale structure;
- the origins of the cosmic acceleration;
- the nature of the dark matter thought to pervade the Universe;
- the formation of galaxies and clusters of galaxies.
Areas of expertise
The cosmology group specialises in the areas detailed below.
Cluster and galaxy formation
Understanding the formation of galaxies and clusters is a crucial part of cosmology, connecting our observable Universe to the underlying dark matter and dark energy.
Galaxy formation plays a central role in modern cosmology as many cosmological probes involve galaxies, from mapping their spatial distribution to counting galaxy clusters, to studying the distorting effect of gravitational lensing on the galaxies by the intervening dark matter. On large scales, we expect galaxies to trace the underlying dark matter distribution. We can also use lensing to infer the "clumpiness" of dark matter around and in-between galaxies. Measuring the galaxy and cluster distribution across cosmic time also helps us measure the growth of large-scale structure in the Universe, as the dark matter collapses under its own gravity, building up larger and larger structures such as clusters.
The Universe also contains “normal” matter, or baryons, which is a complex mixture of galaxies and intergalactic gas. Galaxies form from this gas (which is mainly hydrogen and helium), but also return material in the form of energetic winds and jets, powered by exploding stars and super-massive black holes. Understanding the role of this “feedback” is crucial to our understanding of galaxy formation as a whole, and cosmology is now reaching a level of precision where we must take these baryonic effects into account.
Our research
At JBCA, we use detailed computer simulations to study how galaxies and clusters form with our main aim being to improve our understanding of baryonic physics in cosmology. Our simulations start with realistic cosmological initial conditions, contain both the dark matter and baryonic components, and include the effects of dark energy on the expansion of the universe.
We include both gravity and hydrodynamics in our calculations, as well as model the key astrophysical processes associated with galaxy formation: gas cooling and heating; star formation; growth of super-massive black holes and feedback from massive stars and active galactic nuclei. We compare our simulations to a range of observational data, from radio through optical/infrared to X-ray wavelengths, and are involved in a number of observational programmes. Our work is particularly focused on galaxy clusters, the most extreme environments for galaxy formation and also where the most massive galaxies can be found.
Cosmic microwave background
Measurements of the cosmic microwave background (CMB) temperature and polarization anisotropies have undoubtedly taught us a lot about the Universe we live in, allowing us to pin down the key cosmological parameters to unprecedented precision.
However, the CMB contains another invaluable piece of information that will become available in the future: its frequency spectrum. From observations with COBE/FIRAS we know that the average (all-sky) CMB spectrum is extremely close (better than 1 part in 10,000) to a blackbody with a temperature of 2.726 K. This measurement was awarded the Nobel Prize in Physics 2006 and already rules out many non-standard cosmological models.
However, this is not the end of the story, since even within the standard cosmological paradigm several processes exist that should inevitably lead to tiny deviations from a perfect thermal spectrum, far below (around 100–1,000 times) the sensitivity of COBE/FIRAS but within reach of present-day technology. These spectral distortions will tell us about processes in the early Universe that affect the thermal history due to early energy release or photon production.
CMB spectral distortions
By measuring these distortions, we will open a new, unexplored window to the pre-recombination (redshift z > 1,000), high-redshift Universe. This could teach us more about the small-scale fluctuations created by inflation at scales inaccessible by another means. Eventually, we should also be able to detect the faint, redshifted recombination lines from the hydrogen and helium plasma emitted some 400,000 years after the Big Bang. This distortion can be predicted with very high precision and any deviation from this recombination fingerprint directly implies non-standard physics. The formation of structures and ignition of the first stars during reionization will furthermore heat the cosmic matter and, through Compton up-scattering of CMB photons, create a distortion that will be a large signal for future spectrometers.
Researchers at JBCA pioneered some of the most detailed calculations for these processes, highlighting the range of signals that could be expected (see Figure 1 below). The flexible thermalisation COSMOtherm was developed specifically for this purpose and currently new extensions are being developed to cover an even wider range of scenarios. This work is crucial in the design of future CMB spectrometers as it provide clear targets in terms of sensitivity and spectral coverage.
Future CMB spectroscopy will also allow us to constrain non-standard physics. Energy release by decaying or annihilating particles will create CMB spectral distortions. Similarly, the evaporation of primordial black holes, dissipation of early magnetic fields and even superstrings, to name a few, could leave their traces in the CMB spectrum. All this highlights the great potential of this new window to the early Universe, a window that complements other efforts related to B-mode polarization searches, which currently are one of the great efforts of the CMB community. Researchers at JBCA are heavily involved developing all of these directions and have stimulated several new experimental initiatives with which they are now involved including space-based concepts like PIXIE and also ground-based efforts such as APSERA.
Fundamental physics
Cosmology is sensitive to a range of length and energy scales not accessible to terrestrial physics experiments and hence, if detailed observational information can accumulated, it can be used to probe fundamental processes not possible in any other way. For example, at early times in the Universe one can possibly probe GUT scale physics through its impact on the dynamics of inflation, whereas in late times one can probe infrared modifications to gravity via their influence on the expansion history of the Universe.
Within the JBCA we are interested in all possible impacts of fundamental physics on the evolution of the Universe, but a the moment we are particularly concentrating on the origins of the cosmic acceleration and the observational consequences of topological defects.
We work closely with our colleagues interested in observations, notably, those working on the Cosmic Microwave Background and Weak Gravitational Lensing to constrain our theories, and also with Prof Apostolos Pilaftsis and Dr Fedor Bezrukov in the theoretical particle physics group.
Intensity mapping
In cosmology, Intensity Mapping (IM) is the name given to mapping the intensity fluctuations of a given tracer of mass on large angular scales.
Proposed tracers include the 21cm line of atomic hydrogen and the rotational lines of CO. Rather than detecting individual galaxies, which takes a long time and requires very high sensitivities, IM is based on mapping the large-scale fluctuations, using the integrated emission from many galaxies rather than the individual galaxies by themselves.
This is much more efficient and means that a modest single-dish telescope can be used. Using a well-known emission line also gives the added advantage of knowing the redshift automatically, since the frequency/wavelength gives the redshift directly. A number of IM experiments have been proposed over recent years.
Strong gravitational lensing
Strong lensing - multiple imaging of a background object by the gravity of a massive foreground object - is used to probe mass distributions in the Universe.
Our research focuses on systems in which our line of sight to a distant galaxy or quasar lies very close to a foreground galaxy. Under these circumstances the gravitational field of the foreground galaxy deflects the light from the background object, producing distorted, magnified, multiple images of the background object. The overall effect is known as strong gravitational lensing.
These systems are useful because they provide magnified views of background objects, allowing us to study objects otherwise inaccessible to today's telescopes. They also give unique information about mass distributions in the lensing galaxy, as they are sensitive to all matter regardless of whether it emits light. Studies of lens systems are also important in cosmology.
Weak gravitational lensing
Weak gravitational lensing is the small distortion of background galaxy shapes by a foreground matter distribution.
This phenomenon is a consequence of the curvature of spacetime by matter and energy, as predicted by Einstein's General Theory of Relativity.
The weak lensing of a large number of background sources by the large-scale structure of the Universe, known as cosmic shear, produces coherent shape distortions which can be used to measure the distribution of matter in the Universe, and hence helps us to study the history of large-scale structure formation.
To date, weak lensing has primarily been done using optical surveys, such as the Canada-France-Hawaii-Telescope Lensing Survey (CFHTLenS) and the Kilo-Degree Survey (KiDS). The JBCA is a key contributor to the Dark Energy Survey (DES), which is an optical survey focussed on learning about the content and nature of dark energy. One of the primary tools used to achieve this goal is weak lensing, and early results from the DES science verification data have already produced promising constraints on the equation of state of dark energy and the fraction of matter in the Universe.
The JBCA is also involved in future high-precision optical weak lensing surveys, such as those that will be performed using the Euclid space telescope and the Large Synoptic Survey Telescope (LSST). These surveys are expected to provide an unprecedented insight into the composition and evolution of large-scale structure.
One exciting area of research being conducted at the JBCA is the prospect of performing weak lensing in the radio. Techniques for performing radio weak lensing are being developed using the SuperCLuster Assisted Shear Survey (SuperCLASS), which is a survey of 1.75 square degrees of the Northern sky using the e-MERLIN telescope array. The aim is to use the techniques developed with SuperCLASS in future high resolution radio surveys conducted with, for example, the Square Kilometre Array (SKA). Also, combining radio and optical weak lensing data can potentially remove some of the systematic observational and astrophysical uncertainties that will place limitations on the individual surveys.