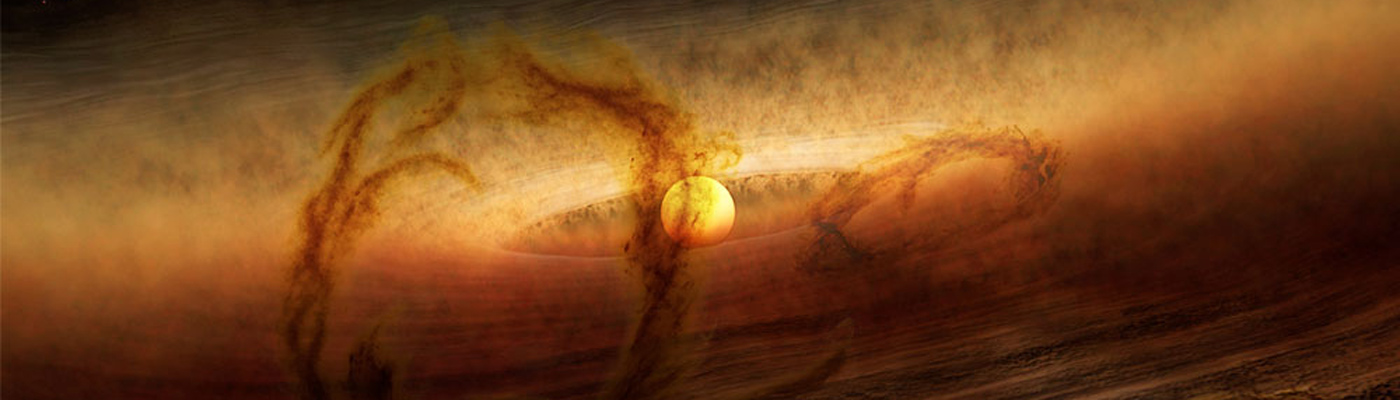
Sun, stars and galaxies
Research in this area focuses on understanding a wide range of astrophysical phenomena using the latest observational techniques, modelling, and theoretical tools.
Our researchers
- Robert Beswick
- Rebecca Bowler
- Philippa Browning
- Chris Conselice
- Clive Dickinson
- Gary Fuller
- Simon Garrington
- Patrick Leahy
- Myfanwy Lloyd
- Andrew Markwick
- Tim O'Brien
- Anna Scaife
- Rowan Smith
- Albert Zijlstra
We cover a wide range of science areas including solar physics, stellar evolution, star and planet formation, and extragalactic astronomy.
We specialise in:
- astrophysical magnetism;
- galactic foregrounds;
- galaxies and active galactic nuclei;
- masers and radiative transfer;
- solar plasmas;
- star formation;
- stellar evolution.
We make observations across the electromagnetic spectrum from radio to X-ray wavelengths, and combine them with theoretical models and laboratory measurements.
Areas of expertise
The Sun, stars and galaxies group specialises in the areas detailed below.
Active galaxies and black holes
Active galactic nuclei (AGN) are the most spectacular of all astrophysical events, capable of outshining the largest galaxies and driving narrow jets of plasma, travelling almost at the speed of light, which can penetrate millions of light-years into the intergalactic medium.
AGN are powered by accretion of material onto the supermassive black holes, massing millions to billions of suns, that lurk at the heart of large galaxies. Although the major outbursts (quasars and radio galaxies) are relatively brief episodes in the life of a galaxy, low-level activity persists in the heart of many galaxies, including our own.
With the exception of dwarfs, all galaxies have the potential to be active and almost certainly went through a period of intense activity at the time when the central bulge and associated black hole were being formed. But only about 10% of local galaxies are classed as active, though the percentage increases as we look to higher redshifts.
Activity is a somewhat slippery concept but is most easily defined as emission resulting from accretion of matter on to the black hole at the centre of a galaxy. Observationally, this activity can manifest itself in the form of Seyfert-like behaviour, ie, re-processed radiation from the accretion disk including strong, broad, high ionisation emission lines and strong blue/UV continuum emission, or as radio emission powered by jet of relativistic particles which can occur either with or without associated Seyfert-like behaviour.
The range of optical luminosities can be enormous ranging from LINERS (poor cousins of Seyferts) to quasars which are the most luminous objects in the Universe. The same is true for the radio emission; the most luminous radio sources are usually accompanied by strong optical activity but the converse is not true.
There really do seem to be two types of AGN, with 'radio loud' AGN producing jets about 1000 times stronger than 'radio quiet' ones, relative to the thermal radiation from the accretion disk.
Most of the research at JBCA focuses on the radio loud objects but even amongst those there is a vast range of luminosities.
Key questions
Some of the key questions are:
- What triggers a galaxy to become active? This is almost certainly related to availability of gas to be accreted by the black hole, perhaps liberated by an interaction with another galaxy, but the details of the process remain a mystery.
- Why are some object radio loud and others, apparently identical in other respects, radio quiet?
- How are the radio jets produced and what regulates the maximum energy of the synchrotron electrons that are accelerated? Radio loud objects emit over the whole EM spectrum up to gamma-ray wavelengths and the study of their spectral energy distributions is an important topic.
- How do radio jets interact with their host galaxies? On a global level it is thought that jets in the dramatic early stages of galaxy formation can regulate the growth of galaxies through feedback. In nearby galaxies such physical process can be studied in detail but at a much less grander scale.
Exciting times are ahead for AGN science: new radio facilities like e-MERLIN, EVLA, SCUBA2 and ALMA are about to come on line and there is a flood of multi-wavelength data from optical/IR/Gamma-ray surveys like SDSS, UKIDDS, Herschel GLAST.
Astrophysical magnetism
Magnetic fields are ubiquitous in space and play a major role in some of the most important astrophysical phenomena.
Unlike the familiar magnetic fields on Earth, magnetic fields in space are not the passive result of electric currents, but are dynamically active, self-generating the currents needed to sustain them through inductive processes in the highly-conducting plasma that fills space.
Without magnetic fields, stars would form in a very different way, there would be no stellar winds, no cosmic rays, no accretion disks or jets in active binary stars and active galactic nuclei, no pulsars and perhaps no neutron stars at all, as magnetic fields seem to be essential to the Type II supernova explosions that form them. Nearly all the complex phenomena on the surface of the Sun are driven by magnetic fields.
Although we know all this, there remains a huge amount to find out about cosmic magnetic fields. We don't know where the seed fields came from that generated the magnetic fields in the interstellar medium of spiral galaxies and the intergalactic gas in clusters of galaxies. Detailed field patterns are poorly known, especially so in our own Milky Way Galaxy, where our view from inside makes it hard to see the big picture. Even in the best-studied cases such as the Sun, the complexity of magnetic phenomena such as reconnection leaves many questions still to answer. For this reason 'The origin and Evolution of Cosmic Magnetism' is one of the Key Science projects for the Square Kilometre Array.
Radio astronomy provides some of the most important tracers of cosmic magnetism, including the Faraday and Zeeman effects which are the best two ways to measure the strength of magnetic fields in interstellar and intergalactic space, and so research into cosmic magnetism drives many research projects at JBCA.
EXPLORE
EXPLORE is a 2 million Euro project funded by the European Commission through the Leadership in Enabling Industrial Technology (LEIT) and Space programme in Horizon 2020. The project is headed by ACRI-ST, an SME which specialises in Earth observation and which has grown from a research company.
The project is developing six novel applications (G-Tomo, G-Arch, S-Phot, S-Disco, L-Explo, L-Hex) that use artificial intelligence (AI) and visual analytics to exploit the vast datasets generated by astrophysics and planetary missions.
Manchester is leading the S-Phot application.
Rather than focus on one main scientific topic, EXPLORE aims to foster synergies between different areas of space science. Four of the applications will leverage data primarily from Gaia, supplemented with data from other surveys, developing tools to help understand the evolution of our galaxy, the 3D distribution of interstellar matter, as well as to support the discovery, classification and characterisation of stars.
The remaining two applications will integrate data from a range of international lunar missions to focus on characterisation of the Moon’s surface and potential human landing sites. A key objective will be to facilitate integration and visualisation of multiple datasets.
In Manchester, Albert Zijlstra and Iain McDonald are developing a tool to combine the GAIA dataset on over 1 billion stars with many other catalogues containing stellar photometry. This will allow accurate determination of stellar temperatures and luminosities, and will allow to find special cases, such as stars with circumstellar disks which are forming planets, or stars which are entering the final phase of their evolution when catastrophic stellar winds remove most of the mass.
Both Masters and PhD projects are available in Manchester on EXPLORE.
Contact Prof. Albert Zijlstra for more information.
Related links:
Galactic interactions and clusters
Galaxies
While the origin of magnetic fields in spiral galaxies is still speculative, a process known as the alpha-omega dynamo amplifies the weak magnetic fields to produce strong large-scale fields through the inductive effect of turbulence and differential rotation.
Nearby galaxies can help us achieve an outside perspective of galactic scale magnetic fields while retaining a decent spatial resolution.
Several observational tools are available to study cosmic magnetic fields with the most useful being radio synchrotron emission. The total synchrotron intensity can be used to estimate the total magnetic field strength. As synchrotron emission is intrinsically polarised, it can be used to determine the ordered component on the plane of the sky. Such polarised emission is mainly seen in regions of low turbulence, specifically in the interarm regions of face-on galaxies.
With edge-on spiral galaxies, the large scale ordered fields in the disks lie plane-parallel along the disk of the galaxy and in the halo they have a X-shaped morphology. This is what is expected from observations of face-on galaxies and their magnetic field amplification by the action of a mean-field dynamo.
We use a variety of telescopes to observe nearby galaxies to help us learn more about the configuration, nature and amplification of galaxies’ magnetic fields and what contribution it has on the ISM. Interferometers such as the Jansky Very Large Array (JVLA) and Low Frequency Array (LOFAR) are commonly used to achieve these goals. Observations at low frequencies with LOFAR make it possible to learn more about the nature of the magnetic field and cosmic ray propagation at furthest reaches of galaxies with little contamination from thermal emission, while higher frequency observations with the JVLA allow us to observe ordered magnetic field through polarisation measurements.
Galaxy clusters
Galaxy clusters are the largest gravitationally bound structures in the Universe. They form at the intersection of filaments in the cosmic web. Galaxy clusters were first discovered in the X-ray, as the hot intra-cluster medium (ICM) emits bright Bremsstrahlung radiation. Despite how bright galaxy clusters are in the X-ray, only about 5% of their mass is contained in the gas. The rest of the mass is contained in Dark matter.
We use radio observations to reveal information about galaxy clusters that is not revealed at other wavelengths. Radio observations look at synchrotron radiation, which trace relativistic electrons in micro-gauss magnetic fields. Large scale diffuse radio emission found in some clusters points to the fact that magnetic fields are present on cluster wide (sometimes Mpc) scales. These observations let us investigate how they might have formed, and how the magnetic fields can influence the evolution of galaxy clusters.
Multi-wavelength surveys
Multi-wavelength surveys allow astronomers to map the sky in different bands, providing a different view of the Universe. Surveys are often focused on answering particular astrophysical questions, but are becoming a vital tool for the community, which can be used for years to come.
Mapping the sky at different frequencies/wavelengths, in different emission/absorption bands, and in polarization, allows astronomers to get a complete picture of the Universe. JBCA is involved in a number of large surveys, covering the entire electromagnetic spectrum.
Research activities
We are involved in a number of sky surveys, which allow us to study a range of astrophysical phenomena across a wide range of wavelengths.
Solar plasmas
"Plasma", consisting of a sea of positively-charged ions and negatively-charged electrons, is sometimes called the fourth state of matter, and more than 99% of the matter in the universe is in the plasma state.
We research plasma processes in the Sun and beyond, developing mathematical models of the complex interactions between plasmas and magnetic fields.
Some key research questions are:
- How is plasma in the solar corona heated to temperatures of millions of degrees?
- How does the process of magnetic reconnection work in the solar corona and elsewhere?
- What is the origin of high-energy charged particles in solar flares?
We are also involved in modelling the interaction of plasma with magnetic fields in magnetically-confined fusion devices such as spherical tokamaks.
Our research
A central theme of our research is modelling the nature and effects of magnetic reconnection in the solar atmosphere.
Magnetic reconnection is a process of fundamental importance in astrophysics, with relevance ranging from galactic magnetic fields, to stellar and solar activity, to planetary magnetospheres, as well as in magnetically-confined fusion plasmas.
Reconnection restructures magnetic fields and provides an efficient means for converting magnetic energy into thermal energy and particle kinetic energy – thus, it plays a central role in solar flares and coronal heating.
Our knowledge and understanding of the solar atmosphere has been revolutionised in recent decades by a wealth of observations, especially from space observatories such as Solar Dynamic Observatory, RHESSI, IRIS and Hinode.
However, new observations have increasingly revealed the complex and dynamic nature of solar magnetic activity, and significant advances in theory and modelling are required in order to understand the physical processes.
A particular challenge for theory is the vast range of spatial scales – from global scales of the order of Mm, which can be well treated using a fluid approach, down to plasma scales, such as the ion gyro-radius, of the order of m or less, which require kinetic models.
Our research thus utilises both magnetohydrodynamic (fluid) models and kinetic plasma approaches, and we are currently developing new techniques to bridge the gap between fluid and kinetic scales. Our modelling involves both large-scale numerical simulations and analytical calculations.
Solar flares
Solar flares are dramatic and complex events which are of interest both in their own right and because of their significant effects on the Earth's space environment by shaping "space weather".
A solar flare involves the release of up to 1025 Joules of stored magnetic energy, in timescales of minutes - hours, with signatures across the electromagnetic spectrum. There is now a considerable body of evidence demonstrating that magnetic reconnection is the fundamental process of energy release in solar flares. However, many mysteries remain unsolved.
One challenge is to explain the origin of the large numbers of high-energy charged particles which are observed, and we are developing models to tackle this question.
A major unsolved problem
Explaining how solar coronal plasma maintained at temperatures of millions of degrees Kelvin, while the surface temperature is only a few thousand degrees, is a major unsolved problem in astrophysics.
A promising candidate for effective dissipation of stored magnetic energy is magnetic reconnection, the same process which is responsible for the energy release in large-scale solar flares.
It has thus been proposed that coronal heating is due to the combined effect of many small flares, known as nanoflares.
We are currently involved in modelling heating by nanoflares, focusing on energy dissipation in twisted magnetic fields.
Over recent years, we have pioneered models of heating in twisted magnetic flux ropes triggered by the ideal kink instability, with application both to understanding confined flares and to coronal heating.
Recent work has focused on predicting the observational signatures of heating in twisted fields, considering both thermal (EUV) and non-thermal emission (Hard X-rays, microwaves).
Star and planet formation
Stars are the building blocks of the universe. They form in the coldest, densest regions of the most massive objects in galaxies, giant molecular clouds.
These molecular clouds contain up to a million times the mass of a typical star, but during their lifetime only about 2% of this mass will turn in to stars. However, once formed these stars drive the evolution of their galaxy.
The formation of stars is a process that has shaped the Universe through cosmic time. Stars created all the elements apart from hydrogen and helium that make up the world we see around us.
When massive stars explode the energy and elements they enrich the surrounding gas controls how galaxies evolve.
Within galaxies, when individual stars form, planets like our own may also be formed around them.
For these reasons, understanding how stars form is an active topic research at the Jodrell Bank Centre for Astrophysics.
Star formation and accretion through cosmic time
The two of the most important processes in the evolution of our Universe are star-formation and accretion. These two symbiotic processes shape the evolution galaxies and what we observe in the Universe today.
How star-formation and accretion interact and affect how galaxies evolve in both the local and distant Universe remains a critical open question in astrophysics.
At JBCA our research focuses on using observations across all wavelengths to understand the astrophysical role of star-formation and accretion processes in galaxies from the local to the distant high redshift Universe.
Studying our galaxy
Studies of objects and processes in our own galaxy can tell us a lot about the formation and evolution of stars across the mass spectrum. But studying our galaxy as a whole is hampered by the fact that the solar system sits within the disc; probing the structure of the Milky Way from this vantage point is not straightforward.
Looking further out into the Universe, the high resolution and sensitivity of telescopes such as e-MERLIN, EVN, ALMA, NOEMA, VLA, Chandra, HST, Herschel and alike can be used to learn much about the detailed structure of galaxies, knowledge which can then be applied to the study of galaxies at much greater distances, as well as to help to determine the evolution and history of our own Milky Way.
Accretion sources
The emission observed from galaxies in both the local and distant Universe is dominated by the processes of star formation and accretion – both fundamental to the formation and evolution of galaxies.
Accretion sources, such as active galactic nuclei (AGN), provide a major power source in the universe and can dominate the emission in objects from distant quasars down to nearby compact stellar mass accretion sources such as X-ray binaries, whereas star formation is a ubiquitous process in all galaxies that enriches the ISM of galaxies.
Feedback from – and between – each of these processes is crucial to our understanding of galaxy evolution and appears to be essential if the observed galaxy luminosity function is to be reconciled with predictions from the standard hierarchical clustering models.
International projects
At JBCA we lead and are involved in a number of large international projects, plus we are actively involved in the development of projects with a number of new facilities, such as the SKA.
Each of these programmes uses leading multi-wavelength observations, with both high and low resolution, to provide detailed diagnostics of star formation and accretion and allow a direct view of the consequences of this process even in the dustiest regions toward the centre of galaxies.