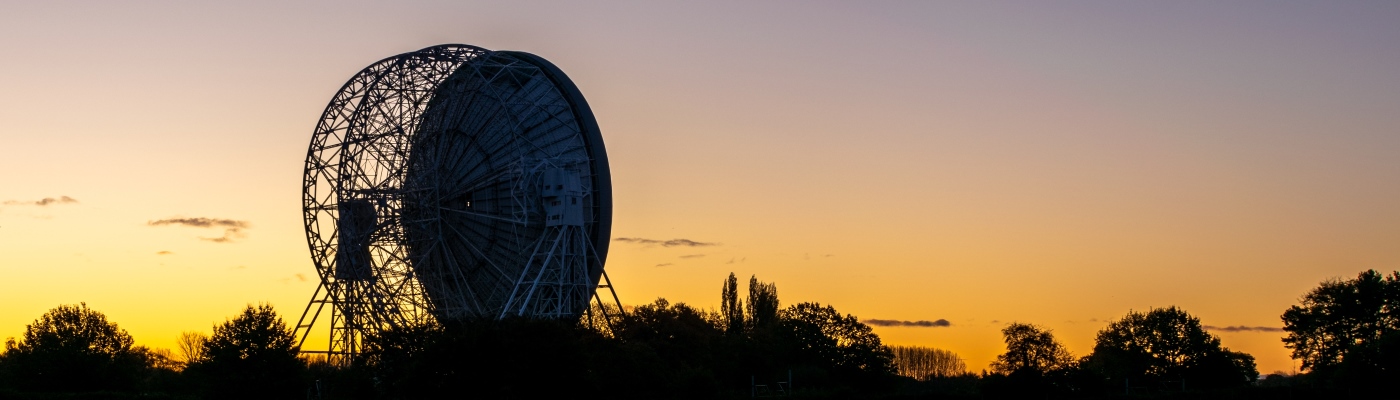
PhD projects
Below is a list of PhD projects being offered in 2025. Please click on a project title to expand it and find out more. You are encouraged to contact prospective supervisors to find out more about their projects.
Our PhD admissions are run on an annual cycle, with most applications due in January, in order to start the programme in September of the same year.
All of the projects listed below are eligible for funding through the general pool of funding schemes listed on our funding page. Any projects marked as "Allocated funding" carry specific guaranteed funding attached to the project. Applicants for "allocated funding" projects will be assessed and shortlisted separately from the general pool. If you have guaranteed funding through a scheme not listed on our Funding page, or are self-funding, please contact our PhD admissions lead, Dr Phil Bull (phil.bull@manchester.ac.uk), directly.
One of our most important funding streams is through the STFC research council, who offer a limited number of fully-funded PhD positions for home and overseas students. For full consideration for STFC funding, your application must be submitted by Friday 24 January 2025. We typically schedule STFC studentship interviews for late February and early March.
Other schemes described in our funding pages typically have application deadlines in early January. If you think one of these would be a good fit for you, please ensure your application is submitted by Friday 3 January 2025 at the latest. For overseas students in particular, it is important to research which funding schemes you may be eligible for, as they all have different deadlines, eligibility requirements, etc.
For details on the application process, please refer to the Postgraduate study page. All correspondence once your application has been submitted will be by e-mail.
When making your application, please include a research statement that briefly outlines any research experience you may already have, and the particular topics you are interested in (1 page max). Students are typically matched with supervisors only after a decision has been made on an offer, and so we only use your list of preferred projects to help guide this matching process. We assess all applications on the same criteria, based on your submitted application materials: academic achievement to date; research aptitude and potential; and the alignment between your goals/motivations and the research environment in the department. Please try to make sure that reference letters are submitted on time, before the deadline.
If you have any questions about the application process, please contact Dr Phil Bull (phil.bull@manchester.ac.uk), directly.
ALMAGAL: The ALMA Study of High Mass Protocluster Formation in the Milky Way
Supervisory Team: Prof. Gary Fuller and Dr Rowan Smith (St Andrews)
Contact: Gary Fuller
Project description: ALMAGAL: The ALMA Study of High Mass Protocluster Formation in the Milky Way
Understanding the formation of massive stars is central to wide range of astrophysics, from the star formation history of the Universe to the physical and chemical evolution of galaxies and the origin of blackholes, pulsars and gamma ray bursts. ALMAGAL is an ALMA Large Programme to observe 1000 young, high mass regions to study the formation and evolution of high mass stars and their associated stellar clusters. This project will involve the analysis of the ALMAGAL observations, and observations from related ALMA projects, to study the properties of the sources detected, the dynamics of the regions and their environments, and the evolutionary status of the protostars. ALMAGAL is large international collaboration and there may be opportunities to spend time working at some of the partner institutions. There will also be opportunities to take part in, and potentially, lead follow-up observations of some of the sources studied with ALMAGAL. The results of the analysis of the ALMAGAL observations will be compared with numerical simulations of massive star formation to help constrain the initial conditions required to form the most massive stars.
Characterising the dynamic magnetospheres of neutron stars
Supervisory team: Dr Patrick Weltevrede
Contact: Patrick Weltevrede
Project description: Characterising the dynamic magnetospheres of neutron stars
Radio pulsars are highly magnetised neutron stars which rotate very rapidly: up to 100s of times per second. During each rotation, the radio emission beamed along the magnetic poles sweeps across the Earth and can be detected by very sensitive radio telescopes as a regular sequence of pulses. The rotation of the neutron stars can be extremely stable, which makes them very accurate clocks allowing tests of the general theory of relativity.
However, for most pulsars, the individual pulses of the observed sequence vary greatly in shape, intensity and polarisation. These variations are caused by largely unknown physical processes in the magnetosphere of these stars. In some cases, these variations happen in a coordinated fashion, which are known as drifting subpulses, indicative of regular dynamical changes in the magnetosphere. On longer timescales (weeks/months/years) the radio emission is known to be correlated to the spin-down rate of the star, showing that the radio emission is linked to powerful magnetosphere-wide processes.
In this project you will explore observational data from the "1000 Pulsar Array" project on the MeerKAT telescope in South Africa (a pre-cursor of the SKA: the Square Kilometre Array, which will be the largest telescope in the world), and Lovell telescope data in Jodrell Bank (one of the largest steerable radio telescopes in the world). In this project you will characterise various types of emission variability seen in the pulse shapes and their polarisation, and explore the implications for magnetospheric theories. Where possible, we will supplement this data with observations from other radio observatories such as the Parkes telescope in Australia (a great instrument, which has discovered more pulsars than any other radio telescope in the world).
CMB spectral distortion anisotropies as a novel probe of cosmology
Supervisory Team: Professor Jens Chluba
Contact: Jens Chluba
Project description: CMB Spectral distortion anisotropies as a novel probe of cosmology
Spectral distortions of the cosmic microwave background (CMB) - tiny departure of the CMB energy spectrum from that of an equilibrium blackbody distribution - have now been recognized as one of the important future probes in cosmology and particle physics. While very challenging to observe, multiple experimental activities have started in the cosmology community with the big goal to improve the long-standing limits by COBE/FIRAS from the 1990s by orders of magnitudes. In addition to the average distortion signals in the CMB monopole spectrum, it has now been highlighted that anisotropic spectral distortions can be directly measured with existing and upcoming experiments (e.g., Planck, Litebird, The Simons Observatory, CMB-S4, the SKA). This directly links distortion science to studies of the CMB temperature and polarization anisotropies, and allows us to constrain new physics related to primordial black holes, primordial magnetic fields, axions, cosmic strings and textures as well as primordial non-Gaussianity.
In this project, you will work on the existing cosmological thermalization code CosmoTherm to develop novel tools for predicting and analysing spectral distortions signals (both average and anisotropic) in light of future CMB missions and experiments. This will identify novel methods for studying early-universe and particle physics in regimes that otherwise remain inaccessible. You bring a keen interest in theoretical physics / cosmology and experience with various modern coding languages (e.g., C++ and Python). The specifics of the project are open and multiple exciting possibilities are available, depending on the student's inclinations and strengths.
CMB spectral distortions meet 21cm cosmology
Supervisors: Professor Jens Chluba, Dr Phil Bull
Project title: CMB spectral distortions meet 21cm cosmology
Spectral distortions of the cosmic microwave background (CMB) are tiny departures of the CMB energy spectrum from that of an equilibrium blackbody distribution. These signals can be used to directly probe the thermal history of our Universe in regimes that are inaccessible by other means. However, CMB spectral distortions can also affect the formation of the 21cm global signal and their fluctuations, which are targeted with dedicated 21cm experiments such as HERA and the SKA. In particular, the presence of distortions in the radio bands can significantly affect the 21cm signal, an aspect that has only recently been explored in more detail.
In this project, you will study how the 21cm signals are generally affected by the presence of CMB spectral distortions. You will study the effects on the evolution of the average spin temperature and also various heating mechanisms, with the goal to develop new tools for connecting CMB spectral distortion physics with 21cm cosmology. A detailed understanding of radiative transfer and coupling effects will be developed both for the late and early evolution of the 21cm signals. The outcomes of this project should be highly relevant to the interpretation of existing and upcoming 21cm experiments and various forecasts will be carried out.
Commissioning the RHINO 21cm global signal experiment prototype at Jodrell Bank
Supervisor: Dr Phil Bull
Contact: Dr Phil Bull
Project description: Commissioning the RHINO 21cm global signal experiment prototype at Jodrell Bank
Early in the Universe's history, before the first stars and galaxies had formed, the only significantly detectable EM radiation came from neutral hydrogen, which has a spin-flip transition deep in the radio part of the spectrum, at a rest-frame wavelength of 21cm. As galaxies began to switch on, the neutral hydrogen was heated and eventually re-ionised. By charting the brightness temperature of the 21cm line over time, we can learn about the magnitude and timing of these early heating processes, and thus learn about the very first stars and galaxies via their impact on their local environment.
The 21cm line is redshifted according to when in cosmic history its emission took place. To probe the time before the reionisation of the Universe, we must observe frequencies in the 70 - 100 MHz range, corresponding to emission from less than a billion years after the Big Bang. Observing this radiation is difficult however; it is faint, while other radio emission processes (such as Galactic synchrotron) occur nearby and can be several orders of magnitude brighter. Radio interference from human activity is also problematic in this part of the spectrum, e.g. FM radio. Instruments designed to observe the 21cm "global" signal in this range (its average over the whole sky) must therefore be calibrated extremely accurately, in order to make it possible for these spurious sources of emission to be subtracted from the data to uncover the 21cm signal itself.
In this project, you will work on designing and commissioning a new 21cm global signal experiment called RHINO, which currently exists as a scaled-down prototype at Jodrell Bank. The main RHINO telescope will be an extremely large (~15m high) horn antenna, which has excellent rejection of many of the systematic effects mentioned above, but will require a lot of infrastructure to build. The prototype is much more manageable however, at only ~3m in height, and can observe at ~350 MHz. The aim of this project is to demonstrate successful science observing with the prototype. This will require developing or refining some components of the receiver hardware, developing a statistics-based calibration pipeline, and observing and subsequently analysing seasons of data from the prototype telescope. The results of this project will then feed into development of the full-sized antenna. (Note: Existing knowledge of electronics/RF engineering is not necessary for this project.)
Cosmology and the dark sector with Euclid weak lensing
Supervisors: Christopher Duncan, Michael Brown
Project title: Cosmology and the dark sector with Euclid weak lensing
Light propagating from distant galaxies has its path bent by the presence of intervening matter, imposing a distortion on their observed shape. By measuring the shapes and positions of a large sample of galaxies across the sky, we can statistically infer knowledge on the properties of the Universe, such as the presence and distribution of dark matter and dark energy: forms of matter, which only interact with light through gravity and are therefore not directly observable. The use of such measures has developed over generations of galaxy surveys designed to utilise this “weak gravitational lensing” signal. The analysis of current data-sets have indicated a tentative disagreement in the preferred Universal model to that determined from the analysis of relic radiation from the early Universe, which may hint at new physics.
In 2023, the Euclid satellite was launched to undertake a survey of billions of galaxies to enable these measurements to unprecedented precision. This will enable a highly precise and accurate inference of the cosmological model of the Universe. The quality and quantity of this data means that its modelling and statistical analysis must be understood better than ever before, including the confounding astrophysical, statistical and optical effects that could induce systematic errors causing us to misinterpret the cosmological signal.
The first data will be released publicly in 2026 alongside a collection of papers analysing this data, with a first release to members of the Euclid consortium in 2025, and subsequent data releases following in the coming years.
The JBCA cosmology group is heavily involved in the weak lensing science analysis for Euclid. In particular, Duncan is co-coordinator of the Euclid key project to extract weak lensing cosmology measurements from Euclid’s first data release.
In this PhD, you will work within the Euclid consortium to contribute to the analysis of Euclid data in the context of an accurate and precise cosmological model for the first data release and beyond. To do this, you will develop techniques for the accurate and precise interpretation of weak lensing observables, including testing the sensitivity of the measured signal and model parameters to known systematic effects, testing their impact on the inferred cosmological model, and developing and implementing methods to mitigate these. This project will involve developing new and existing codes for the analysis and scientific interpretation of large cosmological datasets. Excellent computational and analytic skills will therefore be required.
Deploying and commissioning a state-of-the-art CMB telescope in Chile to study the early Universe
Supervising team: Michael Brown, Angiola Orlando, Mark McCulloch
Project title: Deploying and commissioning a state-of-the-art CMB telescope in Chile to study the early Universe
Simons Observatory (SO) is a new Cosmic Microwave Background (CMB) Observatory located in the high Atacama Desert in northern Chile. It is the premier CMB facility in the world. Its primary objective is to search for a signal, originating from the very early Universe (~10^{-34} seconds after the beginning of the Universe). A detection of this signal (termed "primordial B-modes") would represent a phenomenal breakthrough for fundamental physics. There is simply no other way to explore physics at these energy scales.
The University of Manchester leads the UK contribution to SO. The UK is providing: (i) two state-of-the-art telescopes for SO, (ii) a UK-based data centre for processing the large data volumes and (iii) a program of algorithm development aimed at turning the raw data from the ~100,000 detectors into higher-level data products and scientific results.
JBCA is building one of the two UK telescope "receivers". These receivers are highly sophisticated scientific instruments used to detect the faint incoming CMB radiation. The main receiver build activity is located at Jodrell Bank Observatory (JBO), ~20 miles south of Manchester. With many components already procured, the project is now embarking upon an intensive campaign of receiver assembly and testing at JBO. At the same, two dedicated test systems are under development in the astrophysics labs, located within the Turing building in Manchester. These systems will be used to test and refine two key technologies to be deployed on the UK telescopes - one cryostat will be used to test the continuously rotating half-wave plate, which is used to reject unwanted (non-cosmological) signals from the incoming radiation; the other will be used to validate the performance of the state-of-the-art cryogenically-cooled detectors, which operate at a temperature of 0.1 Kelvin (colder than deep space!).
By the start date of this PhD, the telescope assembly and testing phase of the project will be well underway. The student will work as an integral member of the JBCA SO instrument team (currently consisting of ~10 people). Using the test systems at Turing, they will validate the performance of the rotating half-wave-plate and detector technologies, confirming their readiness for integration into the main receiver system at JBO. They will also become a key member of the team assembling and testing the main receiver at JBO. Once the receiver has been fully validated, it will be disassembled and shipped to the observing site in Chile. The student will join the SO:UK deployment team, travelling to the observing site in Chile to deploy and commission the receiver on its purpose-built telescope mount. The deployment and commissioning phase is currently scheduled to be complete before the end of 2026. The student may also participate in the deployment of the second UK telescope receiver.
During the second half of the PhD project, the student will work with the instrument and data centre teams to analyse the first data from the new UK telescopes. This phase of the project will initially focus on solving the inevitable teething problems that arise with deploying a new scientific instrument. Towards the end of the project, the student will have gained a thorough understanding of the SO:UK telescopes and will be using this unique knowledge to convert the raw data into leading constraints on the physics of the early Universe.
The project will be a mixture of hand-on experimental work and computer-based data collection and analysis. For the deployment and commissioning phase, candidates must undergo a medical assessment for working at extremely high altitude (5,200m) and they must be prepared to deploy to Chile for an extended period of time (up to 6 weeks at a time).
Expanding the LeMMINGs survey using the VLA to study Active Galactic Nuclei and star formation
Supervisor: David Williams-Baldwin
Project title: Expanding the LeMMINGs survey using the VLA to study Active Galactic Nuclei and star formation
Active Galactic Nuclei (AGN) are the central regions of galaxies that include an accreting super massive black hole. While many large black holes are found to produce radio jets that extend into the Inter Galactic Medium, it is the less powerful low-luminosity AGN (LLAGN) that numerically dominate the black hole population. However, LLAGN are much harder to find due to their low bolometric luminosities and smaller scale jets, necessitating high angular resolution and highly sensitive telescopes to not only detect but resolve the emission into individual jet components. The Legacy e-MERLIN Multi-band Imaging of Nearby Galaxies survey (LeMMINGs) was designed to study a statistically-complete sample of low-luminosity AGN in nearby (d < 110Mpc) galaxies, resolving the jet and star formation emission from the compact radio core at the heart of the AGN.
The LeMMINGs project has now been conducted at 1.5 GHz (Baldi et al 2018, 2021a, 2021b) and new results at 5 GHz are being prepared for publication (Williams-Baldwin et al, in prep.). However there is a need to improve the sensitivity of the data to larger scale structures to detect larger scale jets and star forming regions, combining this with the e-MERLIN data to provide a more in-depth study of these LLAGN. The sample is >70% complete with the Very Large Array, probing angular scales slightly larger than e-MERLIN. This project will involve analysing the VLA data on the sample and combining it with the e-MERLIN data to provide a multi-resolution view of LLAGN jets and star formation in nearby galaxies. The candidate should be able to use python and have an interest in radio astronomy on AGN and star formation.
Discovery and Study of the First Galaxies and Stars with the James Webb Space Telescope
Supervisor: Professor Christopher Conselice
Contact: Christopher Conselice
Project description: Discovery and Study of the First Galaxies and Stars with the James Webb Space Telescope
Since its launch in late-2021 the James Webb Space Telescope has started a revolution in our understanding of the first galaxies and stars formed within 500 million years after the Big Bang. This update to the Hubble Space Telescope will observe for the first time the birth of galaxies in the universe. We will also observe some of the earliest stars when they explode as supernova and those seen as gravitationally lensed objects. I am co-leading a JWST guaranteed time observations (GTO) team who will obtain some of the earliest data from JWST for this project, in which these first galaxies and stars will be located and studied.
The student working on this project will lead the discovery of the first galaxies, and studying their properties including their masses, sizes, structures, and merger histories. We are currently obtaining ancillary data with the Hubble Space Telescope and the Very Large Telescope (VLT) in Chile. The student working on this project will take on a leadership role in investigating the stellar populations, ages, structures, and star formation rates of the first galaxies and stars using JWST imaging and spectroscopy. These observations will be interpreted in terms of theories of galaxies formation to test and exclude different ideas for how the first generations of galaxies and star formed.
Faraday rotation and the 3-D magnetic field structures in AGN jets
Supervisors: Dr Emmanuel Bempong-Manful, Prof Rob Beswick and Dr Paddy Leahy
Project title: Faraday rotation and the 3-D magnetic field structures in AGN jets
It is widely accepted that magnetic fields play a key role in the bulk acceleration and propagation of jets produced in active galactic nuclei (AGNs) (e.g., Abstract, Polarization of AGN Jets and Polarization Observations of AGN Jets: Past and Future). However, determining the magnetic field structures in AGN jets from polarisation observations is not always straightforward – due to stratification, radiative transfer, among other factors. Whereas the synchrotron radiation polarisation corrected for Faraday rotation is determined by the structure of the magnetic field in the emitting regions, Faraday rotation on the other hand is produced by magnetic field in thermal plasma between us and the emission, almost always in front of the synchrotron emission rather than mixed with it. As a consequence, gradients of rotation measure (RM) are often seen across radio sources, but their relationship to the structure is still very much unclear, because of limited resolution and/or sensitivity of existing facilities. Thus, there exist open key questions in jet physics including; (1) What are the three-dimensional structures of powerful jets? Do they have highly relativistic “spines”? and (2) What are the magnetic field configurations immediately surrounding jets? Is there evidence for confining fields?. Thanks to the wide-band capability of e-MERLIN (an SKA pathfinder instrument) we can now get excellent signal-to-noise ratio, working around interference – allowing us to determine the variation of RM across a jet at high spatial resolution in a single observation, something which has never been possible previously for powerful jets.
To this end, the e-MERLIN Legacy programme on radio jets has been mapping a number of powerful radio galaxies and quasars to obtain the best possible information about the inner structure of the jet outflows. One target of special interest is 3C 273 – the first documented object to be identified as quasar – which lies at a distance of about 700 Mpc, where we have excellent radio and multi-wavelength data. On sub-kpc scales, the jets in 3C 273 is wide and exhibit a rich linear polarisation structure, making it an ideal candidate to study the origin of Faraday rotation and the 3-D magnetic field configuration in AGN jets. In this project the student will map the structure of the inner jets in 3C 273 using the new multifrequency e-MERLIN observations and attempt to investigate the RM and 3-D magnetic field configuration of the jets. The project can be extended by building a model of the radio spectrum in 3C 273, using ancillary data at other frequencies, and by looking at some other sources of similar type.
Galactic radio emission – understanding our Galaxy for future cosmology missions
Supervisors: Clive Dickinson, Stuart Harper, Vasu Shaw, Paddy Leahy, Jens Chubla
Contact: Clive Dickinson
Project description: Galactic radio emission – understanding our Galaxy for future cosmology missions
JBCA has been at the forefront of studying diffuse Galactic radio emission since the very first days of radio astronomy. We've mapped the entire sky at low angular resolution with several "low" radio frequencies (< 5 GHz) and "high" radio frequencies (30-900 GHz) with the Planck space mission. However, we still do not have a full understanding of Galactic emission across the radio/microwave bands. There are many unanswered questions, including what causes the large radio loops that cover large fractions of the sky or the halo bubbles near the Galactic centre, what is the form of dust/molecules that is responsible for anomalous microwave emission – is it due to spinning dust grains? New polarization observations also reveal a very ordered Galactic magnetic field, both locally and across the Galaxy, but a detailed Galactic model is still missing.
In addition to Galactic science, detailed measurements of the Cosmic Microwave Background (CMB) provide the strongest constraints on cosmological parameters. Future CMB polarization missions are aiming to constrain primordial B modes, caused by a background of gravitational waves, which would be a smoking gun signature that inflation happened in the first fractions of a second of the Universe. However, one of the major challenges is in quantifying and removing "foreground" emission, which for B modes, is at least an order of magnitude brighter than the cosmological signal we're trying to detect!
JBCA is involved in several world-leading experiments that are both trying to measure CMB B-modes and quantify the contaminating foreground emission, including:
- C-Band All-Sky Survey – 5 GHz all-sky survey to map synchrotron intensity and polarized emission with high sensitivity and fidelity, to provide a foreground template for future CMB missions. C-BASS is likely to be the key low frequency data for future missions, as it is at the ideal frequency and is able to map the sky with high sensitivity with minimal systematic errors. We have completed the northern survey and the southern survey will be starting in the near future.
- COMAP Galactic Plane Survey – 26-34 GHz survey of the northern Galactic plane with the COMAP instrument at 5 arcmin resolution to study Galactic emission, particularly AME/spinning dust near 30 GHz. Manchester is leading this sub-project for the COMAP collaboration. The survey is well underway and is expected to be completed ~2025.
- LiteBIRD – next generation Japanese-led space mission to provide the ultimate limits on inflationary B-modes. LiteBIRD is the successor to the immensely successful Planck space mission, to be launched ~2030, and could potentially provide the best limits on inflationary B-modes on large scales (r<0.001). As part of LiteBIRD UK, U. Manchester is responsible for analysis pipelines for component separation and systematic error mitigation.
We are looking for a PhD student that is interested in radio data analysis techniques, with the potential to work on both low level (e.g. improving calibration of C-BASS/COMAP data, data reduction etc.) and high level (e.g. CMB component separation, Galactic science) analyses. The exact nature of the PhD will depend on the experience and interests of the student. Much of the work will be aimed at preparation for the LiteBIRD space mission, including simulations of foregrounds and potential systematic effects. Some of the work may also be applicable to the Simons Observatory (SO) project that Manchester is also involved in.
Graph-PDE-based segmentation of astronomical images
Supervisors: Jonas Latz (Maths), Anna Scaife (JBCA).
Project title: Graph-PDE-based segmentation of astronomical images
Project description (brief): In this project the student will develop a reconstruction-segmentation method using graph-PDE-based segmentation to separate out the components of astrophysical systems within observational data (Joint Reconstruction-Segmentation on Graphs).
Identifying the first galaxies to light the Universe with Euclid and MOONS
Supervisor: Dr Rebecca Bowler
Contact: Dr Rebecca Bowler
Project description: Identifying the first galaxies to light the Universe with Euclid and MOONS
At the cutting-edge of Astronomy research is the study of the formation and evolution of the first galaxies. Through breakthrough observations in the past 30 years it has been possible to identify galaxies from when the Universe was less than 400 million years old. These galaxies have unusual properties compared to the local universe, showing low chemical enrichment and dust obscuration and irregular morphologies. They also produce ionizing photons that cause the Universe wide phase change called cosmic reionization. The goal of this project is to identify the sources responsible for this fundamental transition using spectroscopic data from two new facilities: Euclid and MOONS.
These observations will allow the first determination of the progress of reionization using the Lyman-alpha emission line as a tracer of the neutral fraction of the Universe. The student will become an expert in the analysis of deep spectroscopy of distant galaxies. There is considerable flexibility in the direction of the project in later years, and the student would be encouraged to apply for follow-up data (e.g. with JWST, ALMA) as well as exploit archival data where available. At the end of the project the student would be in an excellent position to continue working with these next generation facilities.
More details on Euclid: Euclid is an optical and near-infrared space mission. It is primarily a cosmology mission but it will discover many thousands of high-redshift galaxies. As Euclid has a near-infrared camera and spectrograph (like Hubble) it will be able to discover very distant galaxies (z = 7-12).
MOONS is a multi-object spectrograph mounted on the Very Large Telescope in Chile. Because it operates in the near-infrared, it is able to detect Lyman-alpha emission from very high redshift galaxies over many square degrees on the sky.
Long term studies of star formation in M82
Supervisors: Professor Robert Beswick and Dr David Williams-Baldwin
Contact: Dr David Williams-Baldwin
Project description: Long term studies of star formation in M82
Understanding the star formation rates of nearby galaxies is important for both galaxy evolution but also our knowledge of stellar physics. In an ideal world, star formation rates can be estimated from optical light received from a galaxy. However, this is complicated as the optical light from an active galactic nucleus must be taken into account, and large amounts of dust and gas can extinct or absorb optical light, biasing estimates. High-resolution radio observations provide the perfect probe of these different classes of object as radio waves are unaffected by this extinction and absorption. The radio waveband is especially useful as it traces both the thermal emission from HII regions of young massive stars, but also the non-thermal emission from the shells of supernova remnants. Radio observations of star forming galaxies are therefore an excellent independent probe of star formation rates which can be used to calibrate other star formation correlations.
The nearby supernova factory M82 has been intensely studied by e-MERLIN and other radio interferometers over the last 4 decades, owing to the high optical absorption which has prevented study of the supernovae, HII regions and exotic transient objects in this galaxy in optical bands. This project will concentrate on radio datasets of M82 over several decades to continue the long-term monitoring of this source and explore new areas such as widefield imaging now that higher performance computers are available. This project can take several different routes depending on the candidates preferred areas and interests, including e-MERLIN data analysis of similar star forming galaxies in the LeMMINGs survey, 10s mas resolution European VLBI Network data to resolve individual supernova remnants or more in depth coding to search for transients over the course of the last 40 year's worth of observations. The candidate should be able to use python and have an interest in radio astronomy on stellar objects.
Mining the Jodrell Bank Pulsar Timing Data Archive
Supervisors: Dr Michael Keith and Dr Patrick Weltevrede
Contacts: Dr Michael Keith and Dr Patrick Weltevrede
Project description: Mining the Jodrell Bank Pulsar Timing Data Archive
The pulsar group at Jodrell Bank has been studying pulsars for over 50 years, and regularly observes around 800 pulsars. These datasets usually stretch back to the discovery of the pulsar, and are therefore the most complete records of pulsar arrival times in the world. Using these data we can track the rotation of the pulsar, and in most cases this means that we unambiguously know when every rotation of the pulsar occurred since it was first observed.
These data are a valuable tool for understanding the complex physics that governs the rotation of pulsars. In particular, it is of great interest to understand how pulsar rotation evolves over time, and how we can characterise and understand the rotational instabilities in the pulsar. We also can use the data to track the position of pulsars over time, and hence get some understanding of the velocity distribution of the pulsars, which we can use to understand the processes leading to the birth of neutron stars.
In this project you will be tasked with extracting deeper understanding of pulsars by applying modern data science techniques such as Bayesian Analysis and Gaussian Processes to the Jodrell Bank pulsar timing database.
Modelling the spectral energy distribution of radio-loud AGNs at z < 1
Supervisors: Prof Rob Beswick and Dr Emmanuel Bempong-Manful
Project title: Modelling the spectral energy distribution of radio-loud AGNs at z < 1
Feedback processes from active galactic nuclei (AGNs) are implicated in current structure formation models to explain for example, the observed number density of galaxies as a function of stellar mass and redshift, and therefore understanding the physical properties of AGNs is key to our understanding of how galaxies and large-scale structures form and evolve over cosmic time. However, the characteristic broadband emission mechanisms in AGNs implies that their spectra tend to be dominated by different specific emission or radiative processes that affect the light as it travels through the ISM/IGM. The spectral energy distribution (SED) of AGNs (e.g., [1],[2],[3]) consequently contains a wealth of information about the host galaxy properties.
In this project we will use radio data from the LOFAR Two Metre Sky Survey (LoTSS; [4]) to model the SEDs of a selected sample of radio-loud AGNs at z < 1 with complementary data at optical and X-ray wavelengths. The goal is to obtain a reliable picture of the physical properties of radio-loud AGNs in the local universe. The student will analyse the observational data (including morphological analysis and pixel-by-pixel SED fitting) in the sample to recover the physical parameters of AGNs and to establish a robust connection between theoretical predictions and observations.
References
Probing environmentally driven galaxy evolution in the last 10 billion years with MeerKAT
Supervisory team: Dr Julia Healy & Prof Rob Beswick
Contact Julia Healy (julia.healy@manchester.ac.uk)
Project title: Probing environmentally driven galaxy evolution in the last 10 billion years with MeerKAT
The environment in which a galaxy resides has long been known to have an impact on how the galaxy evolves. The denser the environment, the stronger the influence. Clusters of galaxies, which are some of the densest known cosmic environments, provide a unique opportunity to study the ongoing environmental processes influencing galaxy evolution. The dominant processes at play in the cluster environment tend to strongly impact the neutral hydrogen (HI) gas disks of galaxies as the HI disk often extends far out beyond the stellar disk making it susceptible to environmental processes before the stellar component of the galaxy is affected, and thus a useful tracer of the environment-driven galaxy evolution. HI is raw fuel for star formation which makes it an important observable in understanding how a galaxy evolves.
Many studies have focused on how the HI is affected in galaxies in clusters in the nearby (z~0) Universe, however, to date there is only a handful of studies that have studied galaxy clusters earlier epochs. This project provides an opportunity to study the gas in galaxies in clusters at epochs earlier than what has been studied previously, thus will provide a unique opportunity to understand how environmentally driven galaxy evolution might change with cosmic time.
In collaboration with colleagues at the University of Groningen, and University of Cape Town, we have obtained wide-area survey with MeerKAT that provides a unique opportunity to study galaxy evolution in a variety of different cosmic environments 7-10 billion years (z~0.4-0.5) in the past. In addition to the 9-pointing MeerKAT data which will provide radio continuum and HI information, we have complementary optical imaging and spectroscopy that will aid in characterising both the environment of the galaxies, as well as provide information on the evolutionary state of the galaxies.
The goal of this PhD project will be to use the MeerKAT data in combination with other multiwavelength data to understand how the evolution of the galaxies at this epoch are influenced by their environment. The student will join this multi-national collaboration, and will learn how to calibrate and image the MeerKAT continuum and spectral line data. Depending on the interests of the student, this project will enable them to focus of statistical studies of galaxies as well as detailed studies of single objects. Should interesting objects appear in the dataset, there will be opportunities to follow up such objects with higher resolution radio observations.
Probing the Galactic magnetic field with POSSUM
Supervisory Team: Dr Paddy Leahy (primary), Professor Anna Scaife (secondary)
Contact: Paddy Leahy
Project description: Probing the Galactic magnetic field with POSSUM
Our Galaxy’s magnetic field plays an important role in the interstellar medium, sometimes dominating the local dynamics and rarely negligible. It helps regulate star formation and accelerates some particles to relativistic energy, forming cosmic rays. These processes are not fully understood, and nor is the structure of the magnetic field, which is often described as turbulent although some organized patterns can also be discerned. There are many observational tracers of the interstellar field, but they all have severe limitations, and so we must make progress by using observational clues to guide theoretical and computational modelling.
One of the most important magnetic tracers is Faraday rotation: the plane of polarization of radio waves change with wavelength, at a rate proportional to the integral the magnetic field component along the line of sight, weighted by the free electron density. This can be relatively easily assessed using extragalactic radio sources, which give us the integrated Faraday rotation along the sight line through the Milky Way. Mapping this across the sky gives a weighted 2D projection of the 3D magnetic pattern. The quality of this information is about to be vastly increased by the Polarization Sky Survey of the Universe’s Magnetism (POSSUM), an international project to measure the polarization of radio sources across most of the sky at 800-1088 MHz, using the Australian SKA Pathfinder (ASKAP), which will give a 10-fold increase in sampling of the Faraday rotation pattern. In addition to extragalactic sources, POSSUM will detect polarization from synchrotron emission in the interstellar medium, which in principle gives more direct information about the 3D field structure, although there are challenging instrumental issues that have to be overcome.
The aim of this PhD project is to study the statistical structure of the Faraday rotation and the underlying magnetic field. The primary observational input will be the POSSUM Faraday results: about one quarter of the sky will have been observed in time to use, including fields close to the Galactic plane with a long sight-line through the disk, and at high latitude where we are looking just through the local layer of the Galaxy. You will draw on other observational results as needed, for instance single-dish observations of the Galactic synchrotron emission at short wavelengths, where the polarization traces the field component in the sky plane. You will interpret your results using theories ranging from toy models that can be run on a laptop to the outputs of massive full-disk magneto-hydro-dynamic simulations being run by Rowan Smith and collaborators.
Pulsar Timing Arrays for the detection of Nanohertz Gravitational Waves
Supervisor: Dr Michael Keith
Contact: Dr Michael Keith
Project description: Pulsar Timing Arrays for the detection of Nanohertz Gravitational Waves
In June 2023 the European Pulsar Timing Array (EPTA) and collaborators around the world announced the first evidence for a background of ultra-low frequency gravitational waves from super-massive black hole binaries in the centres of distant galaxies:
A pulsar timing array makes use of high precision (<1 microsecond) timing measurements of the rotation of millisecond pulsars to form a galaxy-scale gravitational wave detector. We can directly detect the gravitational waves through the quadrupolar correlated variations in the arrival times of pulses from the pulsars.
The Lovell Telescope at Jodrell Bank has been observing these pulsars for decades, and these observations are a key part of the EPTA and International Pulsar Timing Array datasets. More recently new telescopes have also started contributing highly sensitive observations of additional pulsars. In Manchester we are continuing to provide data from the Lovell Telescopes, as well as from the MeerKAT telescope in South Africa, an important precursor telescope for the upcoming international Square Kilometre Array Telescope. We are also involved in studying the pulsars themselves, including the effort to better understand the 'foreground' pulsar noise which can mask the signal from the gravitational wave background.
This project will involve working with the observations from the Lovell Telescope and MeerKAT for Pulsar Timing Array work, and developing new data analysis techniques, with potential to explore ways to exploit recent developments in machine learning. The overall goal is to better understand the signals that we see in the pulsar timing array data, leading to a clear and unambiguous detection of the gravitational wave background.
Pushing the Noise Limit: Low Noise Amplifiers for Radio Telescopes
Supervisory Team: Professor Gary Fuller and Professor Danielle George (Department of Electrical and Electronic Engineering)
Contact: Gary Fuller
Project description: Pushing the Noise Limit: Low Noise Amplifiers for Radio Telescopes
Low noise amplifiers (LNAs) are critical components of receivers for radio telescopes. They offer a number of important advantages over competing technologies such as operating at 20K rather than 4K as well as being better suited for use in large scale imaging array receivers. Recent advances in technology have allowed the development of LNAs which operate at much higher frequencies than previously possible.
This is an opportunity to join the Advanced Radio Instrumentation Group in the Departments of Physics and Astronomy and Electrical and Electronic Engineering.
There are four possible areas of research:
- The designing and testing high performance, wide bandwidth, LNAs at frequencies of up to 300 GHz, and beyond, for use in both single pixel and array receivers on the world’s biggest telescopes.
- The design and construction of receivers for current radio telescopes using new generation, high performance LNAs.
- New processes and materials for transistors for future, high performance generations of LNAs.
- Computer-aided LNA design optimisation.
In each of these areas there is the possibility of placements at various collaborating international institutions, including ESO in Germany and Bologna in Italy.
Quantum Noise in amplifiers for radio astronomy
Supervisor: Professor Lucio Piccirillo
Project title: Quantum Noise in amplifiers for radio astronomy
Quantum mechanics dictates a fundamental limit to the noise added by any coherent amplifier, such as a device that amplifies an input signal while maintaining its phase. This quantum noise, when expressed in terms of a noise temperature, is of the order of hv/k. High Electron Mobility Transistors (HEMT) based amplifiers today represent the state of the art in term of low noise amplification for radio astronomy or other fields where low noise amplification is needed like, for example, quantum computing. The best HEMT amplifiers today generate noise in the range to 5 to 10 times the fundamental quantum limit when cooled at cryogenic temperatures - typically around 20K. When these amplifiers are further cooled down - even to around 1K as first demonstrated by our group a few years ago - they do not exhibit a further reduction in noise.
Our group has been active for many years in trying to understand the physics behind this “flattening” of the noise temperature as a function of the physical temperature. We currently believe that the extra noise mechanism is due to self heating happening in the 2deg region of the transistor where amplification is achieved but also power is dissipated. HEMTs are designed not keeping in mind that at cryogenic temperatures the phonons generated inside the device do not have an efficient path to get in thermal equilibrium with the cold finger responsible for the cooling. The current research in our group is addressing this problem trying to find mechanisms to facilitate the thermalisation of the phonons with the heat sink.
The PhD student will be part of our group addressing this problem through the following lines of research:
- Refining the theoretical basis of noise generation versus physical temperature in HEMT transistors;
- Design and realise new measurement systems to characterise HEMT transistors
- Processing of HEMT transistors chips to improve the self-heating
- Full characterisation of HEMT LNA amplifier using improved transistors.
The student will have access to a variety of techniques and instrumentation: HFSS CAD design and simulations; cryogenic systems design, realization and testing; high vacuum and cryogenic techniques; RF measurements. Part of the work involves the usage of the National Graphene Institute where some processing of the HEMT transistors is carried out: etching, evaporation, sputtering, SEM and TEM microscopy, laser and e-beam writing.
Reionization and the First Large Scale Structures in the Universe with Euclid
Supervisor: Professor Chris Conselice
Project title: Reionization and the First Large Scale Structures in the Universe with Euclid
This PhD project will focus on studying the early Universe using the new Euclid space telescope. In the early Universe, while galaxies were forming, most of the mass existed as neutral hydrogen, making the Universe relatively opaque to energetic photons. Today, however, the Universe is ionized, indicating that a process of reionization occurred, converting neutral hydrogen into ionized gas. This transformation allowed energetic UV light to travel freely through space. Although we know reionization happened, the exact details of how and when it occurred remain unclear. The Euclid Space Telescope, now in operation, offers the opportunity to explore this reionization process on the scale of galaxies and the large-scale structures they inhabit. As part of this PhD, the student will identify and study the largest structures in the early Universe, from the first few hundred million years after the Big Bang. The project will involve using Euclid's spectroscopy to measure the radiation from these structures and determine how and when reionization "bubbles" formed. In essence, the goal is to map the early geometry of the Universe’s reionization, tracing how it progressed from dense, energetic regions to lower-density areas where reionization occurred later. This will reveal the process of the last major phase transition in the Universe with implications for galaxy formation, dark matter, and cosmology.
Relativistic irradiation in spider binary pulsars
Supervisor: Rene Breton
Contact: Rene Breton
Project description: Relativistic irradiation in spider binary pulsars
Binary pulsars are formidable physics laboratories, allowing us to investigate fundamental processes due to their extreme nature (density, magnetic field and gravitational field). This is only possible as they offer proxies to measure their physical parameters via multi-wavelength observations. Of particular importance are the pulsar binaries nicknamed after the deadly spiders ‘black widows’ and 'redbacks', which contain a rapidly rotating millisecond pulsar that gradually destroys a low-mass companion. Current observations demonstrate that these particular systems harbour some of the most rapidly spinning and massive pulsars known to us.
The PhD student will contribute to several projects led by the supervisor’s "Spiders Team" which aim to find and characterise new spiders to understand the underlying physical mechanisms to their remarkable properties. On the observational side, the Spiders Team is involved with multiple pulsar searching efforts conducted at optical and radio wavelengths such as surveys conducted by the TRAPUM collaboration with the MeerKAT telescope. In-depth studies of known systems across the electromagnetic spectrum -- in particular in the radio and optical regime -- are then performed in order to feed into models that enable us to determine system parameters such as orbital inclination, masses, and more. This work uses advanced data science techniques such as Bayesian statistics, forward modelling tools such as MCMC and nested sampling, and machine learning. On the theoretical side, numerical modelling of the binary evolution and stellar structure of the companion is another active area of investigation which offers insights about the extreme interactions which led the pulsar to gradually destroy its companion.
The overarching goal of the PhD project will be to shed light on the impact that the pulsar's relativistic irradiation has on the companion's evolution. The student will look into interaction mechanisms for heat to be deposited into a stellar atmosphere (using photons, lepton or ions as the energy carriers) and their effects on the internal structure of such a star. They will then build self-consistent evolutionary tracks that incorporate the pulsar's irradiation feedback to model the population of sources and compare with observations. Opportunities to get involved with other projects conducted by the Spiders Team highlighted above will also be possible.
Reading list on the general spider pulsar binary topic:
Representation learning for radio astronomy in the era of big data
Supervisors: Prof Anna Scaife and Dr Emmanuel Bempong-Manful
Project title: Representation learning for radio astronomy in the era of big data
The use of self-supervised deep-learning to build foundation models that learn useful representations from large volumes of data is being developed across multiple branches of astrophysics. As in other applications of AI, these foundation models are expected to transform how AI is used in astronomy. The latent space representations from these models can either be used directly to understand and examine structures within the data - for example finding rare types of galaxy; or they can be fine-tuned to address specific supervised problems where large labelled datasets are not available - for example, classifying radio galaxies into different types. With next generation ground-based facilities, such as the SKA expected to generate data volumes on the order of PB scale, such AI assisted latent space representation models will become a powerful tool for retrieving detailed information from observations.
In this project the student will explore the use of such representations for radio galaxy data from the Radio Galaxy Zoo (RGZ) project. This work will initially build on the RGZ representation learned in Radio Galaxy Zoo: Towards building the first multi-purpose foundation
model for radio astronomy with self-supervised learning, and the student will look at how to define different structures/clusters of objects within the latent space, as well as how to extract samples of different types of radio galaxy for scientific analysis. Further work may include multi-wavelength / multi-scale follow-up of interesting populations and/or further development of the foundation model paradigm.
Studying the beginning of the Universe with Simons Observatory
Supervising team: Michael Brown, Daniel Thomas, Erik Rosenberg
Project title: Studying the beginning of the Universe with Simons Observatory
Simons Observatory (SO) is a new Cosmic Microwave Background (CMB) Observatory located in the high Atacama Desert in northern Chile. It is the premier CMB facility in the world. Its primary objective is to search for a signal, originating from the very early Universe (~10^{-34} seconds after the beginning of the Universe). A detection of this signal (termed "primordial B-modes") would represent a phenomenal breakthrough for fundamental physics. There is simply no other way to explore physics at these energy scales.
The University of Manchester leads the UK contribution to SO. The UK is providing: (i) two state-of-the-art telescopes for SO, (ii) a UK-based data centre for processing the large data volumes and (iii) a program of algorithm development aimed at turning the raw data from the ~80,000 detectors into higher-level data products and scientific results.
The University of Manchester hosts the UK data centre and we are playing a leading role in the analysis of the first SO data. (Two SO telescopes, focussed on the search for primordial B-modes, are already operational.) Teasing out the cosmological CMB signal from the large amount of data is a very challenging task, requiring sophisticated data analysis techniques. In particular, careful attention must be paid to the possible presence, and then removal, of instrumental systematic signals (i.e. signals in the data that do not originate on the sky.) Working closely with colleagues across the globe, the JBCA data centre and science analysis team are in the vanguard of this effort.
The student will work closely with the JBCA SO data centre and analysis team to process the latest data from the SO telescopes. This is primarily a computational project (so excellent coding skills, such as Python, are required). Analysis tasks will include low-level processing of the data (e.g. implementing and testing different algorithms for removing atmospheric signals from the data), map-making (projecting the time-ordered data into a map of the sky), and extracting the primordial B-mode signal (via its "power spectrum") from the maps. There is also the opportunity for analytic work to help understand the effects of data processing tasks on the sought-after signal.
In addition to the JBCA SO team, the student will be expected to work in collaboration with international colleagues within the global analysis working groups and collaboration structure of Simons Observatory. The student should be prepared to travel to international SO collaboration meetings and workshops as a routine part of this PhD position.
Superconducting Parametric amplifiers for radio astronomy
Supervisor: Professor Lucio Piccirillo
Project title: Superconducting Parametric amplifiers for radio astronomy
Thin films made of superconducting materials exhibit a non-linear inductance caused by the kinetic properties of Cooper pairs and free electrons. When a non linear element is “pumped” with an AC signal, parametric amplification is generated. Our group has been building superconducting resonators optimised to work as narrow-band high gain parametric amplifiers. Being composed exclusively of superconducting material, the resistance of the amplifier is identically zero e this allows the amplifier to work with a near quantum limited noise. Under the proper conditions, sub-quantum noise level can be obtained through the so called “noise squeezing”.
This PhD concern the design, fabrication and testing of superconducting parametric amplifiers. The processing will happen in the National Graphene Institute where the student will be allowed access, after proper induction. Once amplifiers are designed and manufactured, the student will use our sub-K test bed to properly characterise the noise, the gain and other important parameters. These amplifiers will then be used in state-of-the-art astrophysics projects like, for example, our axion search experiment under construction in our laboratories.
SZ Science with the Next Generation of CMB Surveys
Supervising team: Richard Battye, Jens Chluba, Scott Kay
Project title: SZ Science with the Next Generation of CMB Surveys
The Sunyaev-Zeldovich (SZ) effect is the spectral distortion of the CMB resulting from the inverse Compton scattering of CMB photons off free electrons. It is a powerful method for constraining cosmological parameters (e.g. from counting galaxy clusters) as well as measuring the distribution and properties of hot gas in groups, clusters and filaments, where most of the Universe's baryons are thought to reside. Recent CMB surveys using the South Pole Telescope, Atacama Cosmology Telescope and the Planck satellite have produced large samples of clusters and sky maps of the SZ effect.
Over the coming decade, the next generation of CMB surveys such as with the Simons Observatory (SO) and LiteBIRD will vastly increase the SZ data volume, allowing us to measure the SZ effect with greater sensitivity and for much larger samples of objects extending to lower masses and higher redshifts.
The JBCA is playing a leading role in the SO project in particular, and will be involved with many aspects of the SO SZ analysis. Novel new areas include using relativistic effects to probe the gas temperature and velocity structure, and improving SZ models guided by state-of-the-art hydrodynamical simulations. In this PhD project, the student will join JBCA's team of SZ scientists to work on the development of theoretical SZ models and analysis of SZ data. The supervisory team of Battye, Chluba and Kay, together, have extensive and complementary experience in SZ theory, modelling (including simulations) and cosmological data analysis. The student will also have the opportunity to interact with relevant international collaborations on both theory and observational sides.
Testing models of relativistic jets with e-MERLIN
Supervisory Team: Dr Paddy Leahy (primary), Dr Emmanuel Bempong-Manful (co-supervisor)
Contact: Paddy Leahy
Project description: Testing models of relativistic jets with e-MERLIN
Many of the issues in understanding structure formation and black-hole growth are thought to be resolved by suitably-tuned feedback of energy and momentum from AGN activity – from dense to diffuse phases of matter. Relativistic plasma jets of radio-loud AGNs are particularly thought to be responsible for the production of the most energetic photons and hadrons in the observable Universe, thereby playing a key role in this feedback cycle. However, the physics driving the observed jet structure in these cosmic outflows remains an open question. To resolve them we need to quantify the mass, momentum and energy inputs from jets and to work out how they interact with their environment.
Here at Manchester we are leading an ambitious global effort (The e-MERLIN Jets Legacy programme) to resolve these and other key questions in extragalactic jet physics. The programme has been mapping a number of powerful radio galaxies and quasars, allowing us to study for example the detailed structure of magnetic field in relativistic jets (the synchrotron radiation polarisation) and in the foreground gas (via Faraday rotation). Deep LOFAR observations have also been acquired for the programme, providing us with the unique opportunity to probe the dynamics and energetics of relativistic jets over broad frequencies.
The goal of this PhD project will be to utilize these new radio observations to map the jet structure of some well known powerful radio galaxies in order to test the relativistic beaming model in jets. The student will join the e-MERLIN Jets Legacy collaboration and become a part of the LOFAR-VLBI Working Group. Depending on their interests, the project could be extended into a multiwavelength campaign with complementary observations at optical and X-ray wavebands, and/or perform numerical MHD simulations to model the jet kinematics and compare theory with observations.
The Role of Magnetic Fields in the Formation of Massive Stars
Supervisory Team: Professor Gary Fuller and Dr Rowan Smith (St Andrews)
Contact: Gary Fuller
Project description: The Role of Magnetic Fields in the Formation of Massive Stars
Magnetic fields are ubiquitous in the interstellar medium but their detailed role in the formation of stars is as yet unclear. In the dense star forming regions of molecular clouds the magnetic field can be traced through observations of the polarized continuum emission from dust grains which align with the magnetic field. However, to understand how magnetic fields affect the evolution of the gas, observations of the magnetic field must be combined with observations of the cold, molecular gas in the regions. This project will study the impact of the magnetic fields as gas flows from parcsec-scale clumps down to individual star forming cores and protostars. It will involve observations of polarization emission from dust and molecular lines to study the magnetic field and molecular gas covering a wide range of size scales in star forming regions. Part of the project will be developing new methods to compare the polarization and line observations with the results of state-of-the-art magneto-hydrodynamics simulations. This work will be carried out in part as part of the follow-up of ALMAGAL, the ALMA large programme studying the formation and evolution of high mass stars and the ERC Synergy project ECOGAL.
Topological defects at the electroweak phase transition
Supervisor: Richard Battye
Project title: Topological defects at the electroweak phase transition
Topological defects can be formed at cosmological phase transitions where the vacuum manifold has non-trivial homotopy. The objective of this project is to explore the possibility that topological defects might be formed at the electroweak phase transition in extensions of the Standard Model of particle physics. We have been studying the so-called two Higgs-Doublet model (2HDM) which is one of the most popular extension.
The project will involve further developing the work often using large-scale computing resources based in the Manchester elsewhere in the UK. It will also develop the picture of cosmological evolution of such defects, and also consider how such a defect might be produced in a particle accelerator such as the LHC.
Towards 100,000 exoplanets with the NASA Roman mission
Supervisor: Dr Eamonn Kerins
Project title: Towards 100,000 exoplanets with the NASA Roman mission
Microlensing is proving to be the most capable method to find cool low-mass planets, including planets around the most common types of star, and planetary architectures that most resemble that of our own solar system. The demographics of these planets is also crucial for testing planet formation theories.
In October 2026, NASA is expected to launch the $4.3 billion Nancy Grace Roman Space Telescope (Roman), which will undertake a dedicated exoplanet microlensing survey over 5 years. Roman will have a similar sensitivity and resolution to the Hubble Space Telescope, but will have 100x its field of view and 1400x its survey speed. Roman will revolutionize our understanding of exoplanet demographics by discovering up to 200,000 new hot and cool exoplanets over Galactic distances using the transit and microlensing methods. Dr Kerins is the ESA-appointed scientist to the NASA Roman exoplanet survey, and he leads one of the major working groups investigating exoplanet demography with Roman.
At Manchester, we are developing a sophisticated Roman transit and microlensing simulation framework. This is being used to help us understand Roman's capabilities for: mapping out exoplanet demography over Galactic distances; constraining planet formation; detecting and characterising extreme exoplanetary systems, including free-floating planets and exomoons; determining the Galactic abundance of habitable zone planets.
PhD projects are available to undertake studies in one or more of these areas. The projects are computational in nature, requiring using both existing parallelized python codes and developing new ones. We have a 64-core AMD Threadripper machine dedicated to our exoplanet work. The exoplanet group at Manchester, working with Kerins, currently comprises five PhD students and one MSc student. This research will contribute to our group's ongoing contribution as part of the NASA Roman Galactic Exoplanet Survey Project Infrastructure Team.
View-based equivariance in multi-source deep-learning for radio astronomy
Supervisor: Professor Anna Scaife
Project title: View-based equivariance in multi-source deep-learning for radio astronomy
The volume of labelled data available in astronomy for supervised deep-learning applications is very small compared to other fields. However, given the significant volume of unlabelled data available, the potential of self-supervised learning approaches to underpin the use of AI in astronomy is high. This project will look specifically at the use of view-based self-supervised learning (SSL) for building astronomical foundation models. View-based SSL uses cost functions based on the mutual information between the embeddings for different views (augmentations) of the same data sample. The resultant embedding should be a structured, high-level representation of the training data that is invariant to those views.
Since archival astronomy data are likely to be highly heterogeneous with regard to their sensitivity (noise level), distribution of spectral (frequency) information and, in the case of radio interferometers, the range of spatial scales probed, a potential negative consequence of building foundation models from multi-source databases is that they do not learn the joint distributions of causally-relevant features related to the astrophysical systems under observation, but rather the characteristic parameters of the different types of observation (i.e. sensitivity, resolution, observing frequency etc).
In this project the student will examine whether equivariance to observational characteristics can be enforced in astronomical deep-learning models through the use of multi-source views from archival multi-survey data. They will then go on to examine the sensitivity of various model properties such as generalisation, quantification of dataset-shift and fine-tuning to a multi-source training regime, using test data from SKA pre-cursor telescopes such as MeerKAT and ASKAP.