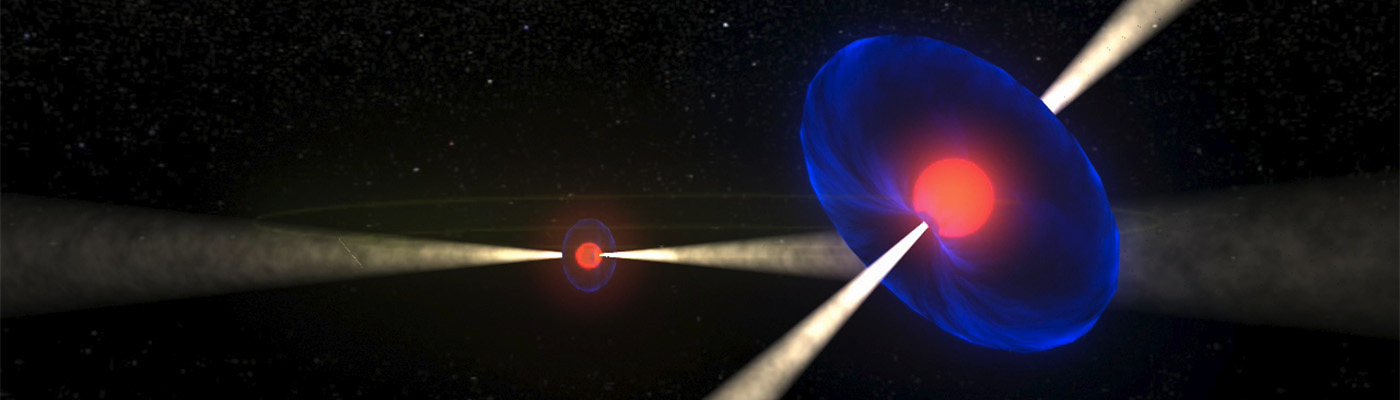
Pulsars, Exoplanets and Transients
We study all manner of sources, they vary as a function of time across the electromagnetic spectrum including: pulsars, fast radio bursts, extrasolar planets, novae and micro-lensing.
Our researchers
- Rene Breton
- Francis Graham-Smith
- Michael Keith
- Eamonn Kerins
- Michael Kramer
- Andrew Lyne
- Tim O'Brien
- Benjamin Stappers (Theme Lead)
- Patrick Weltevrede
The sky is highly variable and studying this variability can provide us with insight into the physical processes which govern the formation, evolution and origin of these sources. We use multi-messenger observations at cutting edge facilities to undertake some key science goals such as:
- the origin of fast radio bursts and their use as cosmological probes
- using pulsars as precision tools for testing gravity and studying: extremes of plasma and magnetism, nuclear equations of state and binary system evolution.
- the structure of our galaxy as determined through microlensing
- the physics of planetary atmospheres
- the physics of explosive events such as novae
We are also interested in next generation telescopes and are involved in the development and science programs for the SKA, EUCLID, LSST and many others.
Areas of expertise
The pulsar, exoplanet and transients group specialises in the areas detailed below.
Binary MSPs
The Double Pulsar, PSR J0737-3039A/B, is the only binary pulsar system in which both neutron stars have been detected as radio pulsars.
This is a rare situation as it requires the electromagnetic beam from both pulsars to intercept our line of sight at the same time. The Double Pulsar was discovered in late 2003 by an international team of astronomers with the 64-m Parkes radio telescope in Australia as part of a high-latitude multibeam survey of the Southern sky. Double Pulsars are one of the research topics of the pulsars and time domain astrophysics group.
Exoplanets and SETI
The detection and study of extra-solar planetary (exoplanet) systems is a relatively new and exciting field of astrophysics. Since 1995 more than 4000 exoplanets have been discovered and the rate of detection is accelerating as methods improve.
One of the principal interests in the search for exoplanets is the possibility of life, including extra-terrestrial intelligence (ETI). JBCA is a leader both in the detection and characterisation of exoplanets and in the Search for Extra-terrestrial Intelligence (SETI).
Exoplanet research at JBCA
At JBCA we are engaged in the detection and study of exoplanets using two complementary detection methods: gravitational microlensing and the transit method. Microlensing is a powerful method for finding cool, low-mass planets that are impossible to find with other current methods. The orbits of cool low-mass planets are not predicted to have evolved much since the time of planet formation, and so microlensing provides a very direct and potentially sensitive probe of planet formation theories. Microlensing is even being used to find so-called free-floating planets - planets that appear not to be bound to a host star. The transit method has been used to discover thousands of hot and warm exoplanets located closer in to their host. These include a number of potentially habitable planets. There are a number of follow-up techniques that can be applied to transiting planets to allow their internal composition to be constrained, their atmosphere to be probed, or even to find evidence for the existence of moons around exoplanets (exomoons).
In the context of exoplanet detection microlensing describes the temporary magnification of background stars by the passage of a foreground planetary system across the line of sight. The foreground host star produces a magnification signal which typically lasts for weeks to months, while its planets may induce brief distortions of this signal lasting for around a day (for a Jupiter mass planet) down to hours (for Earth mass planets).
We have recently used data from the NASA Kepler K2 mission to conduct the first space-based search for exoplanetary microlensing. This includes events that may be due to free-floating planets, planets that are unbound to a host star. The origin, mass and abundance of such planets can provide a crucial 'smoking-gun' signal of the planet formation process.
We have a leadership role in the ESA Euclid Exoplanets Working Group. Euclid is a medium-class ESA mission due for launch in 2022 whose primary science is the study of dark energy. But it is also expected to undertake other additional science activities, including a high cadence near-infrared search for exoplanets towards the Galactic bulge using microlensing. Such a survey would allow the mass, distance and motion of cool exoplanets to be determined down to Mars mass over exoplanet host separations ranging from 0.5 AU out to the free-floating exoplanet regime. This would provide the perfect complement to the hot and warm exoplanet census undertaken by the NASA Kepler transit mission. We have developed world-leading simulations of Galactic microlensing that are being used to design exoplanet microlensing surveys for the ESA Euclid mission and the NASA Nancy Grace Roman Space Telescope. We also lead an international survey team (SPEARNET) that is studying the atmospheres of transiting planets using the technique of transmission spectroscopy.
Manchester is a World leader in simulations of Galactic microlensing and their use in shaping the design of space-based microlensing surveys. Our simulations provide a near perfect match to the observed distribution of microlensing events observed by ground-based surveys like OGLE-IV. We have pioneered new approaches that have led the design of the Euclid exoplanet survey. The same techniques are now also being used to design the exoplanet microlensing survey that will be a primary science objective for the NASA Nancy Grace Roman Space Telescope that is due for launch in 2026.
Members of JBCA are also using the technique of transmission spectroscopy to study the atmospheres of transiting exoplanets. In collaboration with colleagues at the National Astronomical Research Institute of Thailand (NARIT) we have formed the Spectroscopy and Photometry of Exoplanet Atmospheres Research Network (SPEARNET). New ongoing and upcoming transit surveys such as NGTS, TESS and PLATO will be uncovering thousands of relatively nearby exoplanet systems that will be suitable for follow-up using techniques like transmission spectroscopy. We are therefore transitioning from a 'target-starved' era of few suitable targets to an 'asset-starved' era where there are insufficient ground-based telescopes available to follow-up all targets. SPEARNET uses a distributed network of optical telescopes to monitor transits at optical and near-infrared wavelengths. These observations allow a transmission spectrum of the exoplanet atmosphere to be obtained, allowing the study clouds, hazes and alkali metals in the atmospheres of hot Neptunes and Jupiters that point to their internal chemistry. SPEARNET is pioneering an innovative approach of optimised automated selection of targets for study by a large globally-distributed network of telescopes of varying aperture. We are designing new powerful transit fitting codes suitable for multi-telescope, multi-wavelength, multi-epoch datasets. We are also innovating new machine-learning approaches for the retrieval of exoplanet atmosphere models from the observed data. These innovations will provide robust tools for future large-scale transmission spectroscopy surveys such as will be undertaken by the ESA Ariel mission, which is due for launch in 2028.
SETI research at JBCA
There is now a new energy in SETI, brought about through increases in detector sensitivity, advances in data science and philanthropic financial backing. There are two approaches to current SETI strategies. Survey SETI involves surveying large regions of the sky in order to listen out for signals potentially from any civilisation within range of our sensitivity. Sweeping large areas of sky enhances the chances of finding a signal. However, it presents a huge data processing and signal processing challenge that may limit the frequency space that can be covered, or the sophistication of the signal search methods used on the data. Survey SETI is most often carried out at radio frequencies where there is an absence of narrow-band emission from known natural astrophysical sources. Targeted SETI offers a complementary approach in which small numbers of systems are monitored more intensively, either over a broader frequency range, or with dense temporal sampling, or by applying more sophisticated signal search techniques. In this case the targets need to be chosen based on some evidence for their potential as habitable hosts. Targeted SETI is usually undertaken at both radio and optical wavelengths, sometimes targeting known exoplanet systems.
JBCA is at the forefront of radio survey SETI efforts, as partners in the Breakthrough Listen project. Breakthrough Listen is a $100 million ten-year initiative that aims to find evidence for ETI or else place limits on its abundance that are vastly stronger than those previously accomplished. Breakthrough Listen is using a global network of some of the World's most sensitive radio telescopes, including Manchester's Lovell Telescope at Jodrell Bank, to monitor large areas of the sky for evidence of signals indicative of a non-natural origin. As Breakthrough Listen progresses it will also inevitably provide a truly unique probe of many kinds of rare and transient natural astrophysical phenomena.
Recent results from the Breakthrough Listen project are already placing much stronger limits on extra-terrestrial radio transmissions than was possible only a short time ago. At Manchester we have pioneered new approaches for analysing survey SETI datasets that have resulted in a dramatic increase in their effective sensitivity and the strongest limits to date on the prevalence of ETI. But the search parameter space remains vast and so the observational journey is still really only at the beginning.
JBCA is also interested in searching for signs of intelligent life at other wavelengths, in addition to the radio domain. More generally we looking for the imprint of technical civilisations in astronomical data. One example, is the waste heat that could be produced by energy-intensive civilisations - these appear as significant excesses in the infrared emission of stars and galaxies.
As well as survey SETI we also have interests in targeted SETI at radio and optical wavelengths. We are using game theory to advocate smart strategies for the selection of systems towards which SETI should be conducted. We have proposed the idea of Mutual Detectability as a means to identify planetary systems for targeted SETI. The mutual detectability approach is based on the idea that the best systems to target may be those that can most easily observe Earth and find evidence that it is habitable (or even is inhabited). This idea motivates surveys for transiting exoplanets that lie within the so-called Earth Transit Zone, which is a narrow band on the Ecliptic Plane from where civilisations would be able to see Earth transit the Sun.
Fast Radio Bursts (FRBs)
The first example of an FRB was discovered in 2007 in archival data of the Magellanic Clouds taken by the Parkes telescope, Australia, in 2001. Within 480 hours of observations, a lone radio pulse, 5ms long and estimated to contain ~10^33 joules of energy (the total output of the sun over 10 years) was discovered, sparking immense excitement in the astrophysical community.
The current tally of published these mysterious signals still stands at less than twenty (17) and yet the field of FRB research is one of the most rapidly evolving areas of radio astronomy.
Glitches in pulsars
Glitches are sudden changes (usually increases) to the spin rate (the period) of the pulsar. Glitches are usually followed by quasi-exponential recoveries back towards the initial spin rate. The phenomenon is generally more common in younger pulsars.
The exact mechanism by which glitches occur is not well understood however they are thought to be due to the crust of the neutron star rotating at a slow rate compared with the superfluid interior. Occasionally, the interior couples to the inner crust and spins the star up abruptly.
Unmodelled glitches cause the residuals to drift away from zero, following a quadratic signature. Some pulsars never recover their initial spin-rates following a glitch. Less commonly, a pulsar will 'over-recover', ending up with a longer period than it started with.
Novae
Novae are interacting binary systems in which a white dwarf is accreting matter from a companion star, either a main-sequence dwarf or occasionally an evolved star like a red giant.
Nova explosions are caused by runaway thermonuclear reactions in the material accreted onto the white dwarf leading to a sudden brightening at optical wavelengths accompanied by the ejection of matter at speeds of several hundreds to thousands of km per second. The ejected matter is a mix of material from the companion, processed by nuclear reactions and, perhaps, some material from the white dwarf.
The expanding ejecta are often not spherical and it is commonly assumed that this is the result of interaction with the companion star in a common-envelope like phase (although other factors like the magnetic field and spin of the white dwarf cannot be excluded).
Nova systems are excellent laboratories for studying a wide range of astrophysical phenomena including accretion, nuclear reactions, shock waves, jet formation, photoionisation and dust formation. In some cases (when the white dwarf grows in mass as a result of accretion) even providing possible Type Ia supernova progenitors.
Working with colleagues from several UK and international universities, our group has for many years carried out theoretical and observational studies of novae, linking them to planetary nebulae and supernovae, and combining modelling with observation at wavelengths ranging from the radio to the X-ray. The work includes: multi-frequency monitoring of novae in outburst; structural studies of nova ejecta using a combination of imaging and spectroscopy; and hydrodynamic simulations of mass-loss using parallelised numerical codes developed in-house and also applied to PNe and astrophysical jets.
Current work focuses on the use of e-MERLIN and VLBI to image the expanding ejecta and shock waves at very high angular resolution. This is combined with observations at other wavelengths to develop models for the 3-dimensional structure of the ejecta. We are particularly interested in understanding the mechanisms that shape the ejecta, in improving estimates of the mass ejected and answering the question of whether the white dwarfs in some novae will exceed the Chandrasekhar limit and explode as supernovae.
Pulsar emission physics
Pulsar Emission Physics is one of the research topics of the Pulsar and Time Domain Astrophysics group. Pulsars are one of the most stable rotators in the universe and the stability of the rotation of some pulsars is comparable to that of an atomic clock (e.g. Petit & Tavella 1996).
Pulsars spin down gradually due to the loss of rotational kinetic energy into high-energy plasma and electromagnetic radiation. A simple spin-down model based on the rotational frequency and its first time derivative is sufficient to explain their slow-down in general. However, most pulsars show irregularities in their spin properties, mainly due to glitch events (i.e. a sudden increase in rotational frequency; e.g. Espinoza et al. 2011; Yu et al. 2013) and low-frequency timing noise. These spin irregularities are generally seen in pulsar timing residuals, i.e. the difference between the measured time of arrival (TOA) of the pulse from the pulsar at the observatory and the spin- down model predicted TOA.
In a number of pulsars, this behaviour has been correlated with mode-changing - sudden changes to the pulse profile shape. This is an exciting result as this means it is possible to know, depending on observing cadence and signal-to-noise, when these switches occur in the data by looking at the profile shape thereby allowing us to model and remove timing noise from our data effectively improving the precision of a large number of pulsars which display this behaviour. Our long-term data sets of pulsars provide a great opportunity to study such irregularities in general, and then understand the emission physics of pulsars.
Pulsar searching
Notable pulsar research is detailed below.
With GMRT
We are conducting a survey for pulsars and transients using the Giant Metrewave Radio Telescope (GMRT). The GMRT High Resolution Southern Sky (GHRSS) survey is an off-Galactic-plane (|b|>5) survey in the declination range -40 degrees to 54 degrees at 322 MHz. With the high time (up to 30.72 micro-sec) and frequency (up to 0.016275 MHz) resolution observing modes, the 5-sigma detection limit is 0.5 mJy for a 2 ms pulsar with 10% duty cycle at 322 MHz. Total GHRSS sky coverage of 2866 square-deg, will result from 1953 pointings, each covering 1.8 square-deg. The 10-sigma detection limit for a 5 ms transient burst is 1.6 Jy for the GHRSS survey. In addition, the GHRSS survey can reveal transient events like rotating radio transients or fast radio bursts.
With LOFAR
The Low Frequency Array (LOFAR) is a new-generation radio interferometer based in the north of the Netherlands with further stations across Europe. It will operate in the relatively unexplored frequency range 30-240 MHz. With its dense core and long baselines, LOFAR has unparalleled sensitivity and angular resolution in the low-frequency radio regime. LOFAR's capabilities make it an important pathfinder to the Square Kilometre Array (SKA).
With Parkes
The High Time Resolution Universe in the southern sky (HTRU-South) was a survey for pulsars and fast transients, running between November 2008 and January 2014 at the 64-metre Parkes radio telescope in Australia. Combined with its sister survey covering the northern sky, HTRU-North, currently underway at the 100-metre Effelsberg radio telescope in Germany, the HTRU survey is a true all-sky pulsar survey. With some data processing still on-going, HTRU-South has discovered over 200 previously unknown pulsars, including more than 30 of the most rapidly-rotating pulsars, known as millisecond pulsars (MSPs). More about the survey in general, and the low-latitude part in particular, can be found on HTRU-South low-latitude Galactic plane website created by Cherry Ng. The site also contains a list of all published HTRU-South papers and PhD theses.
One of the most interesting of the HTRU MSPs is in a binary system with a companion whose mass is similar to that of Jupiter. It is thought that this companion was once the star which spun up the pulsar, but has now been transformed into a planet over the lifetime of the system. You can read more about this discovery in the University's press release 'A Planet Made of Diamonds'.
Pulsar timing
European Pulsar Timing Array (EPTA)
The European Pulsar Timing Array is made up of scientists and engineers from over 20 institutions across Europe working in observations, instrumentation, data reduction, and theory. By using the 5 biggest radio telescopes in Europe (The 76-m Lovell in the UK, the 94-m equivalent WSRT in the Netherlands, the 100-m Effelsberg in Germany, the 64-m SRT in Sardinia, and the 94-m equivalent Nançay Radio Telescope in France), the EPTA is capable of observing at a wide range of frequencies with high cadence per source.
The LEAP Project
As a means of bridging the gap in sensitivity between the current generation of radio telescopes and the SKA, the European Pulsar Timing Array has found an innovative way to combine their existing telescopes into a fully-steerable virtual telescope, of 195-m equivalent dish size, known as LEAP: The Large European Array for Pulsars.
LEAP is used for monthly observations of millisecond pulsars, where data is recorded simultaneously with The Lovell Telescope, the Effelsberg 100-m Radio Telescope, the Nançay Radio Telescope, the Westerbork Synthesis Radio Telescope, and the Sardinia Radio Telescope. The hard disks containing the observation data are shipped to Jodrell Bank Observatory, where they are put on a work cluster for processing.
The main goal of LEAP is to achieve the high sensitivity required to make a direct gravitational wave detection, but the high sensitivity afforded by LEAP is useful for individual-pulsar science projects. Some projects to be completed in 2016 include a study of giant pulse emission from B1937+21, single-pulses from J1713+0747, and long observations of the galactic centre magnetar J1745-2900.
SKA and next generation instrumentation
The Square Kilometre Array link to is currently being designed by an international team of scientists and engineers. When finished, the SKA will be the world's largest radio telescope, with a collecting area of one million square meters.
The telescope will be co-hosted on two sites, with hundreds of 15-m radio dishes located in Southern Africa and thousands of dipole antennas in Western Australia. This will enable astronomers to observe radio waves at a large frequency range (from 50 MHz to 24 GHz).
The SKA headquarters are located on the site of the Jodrell Bank Observatory, with astronomers and engineers from over the world actively involved in the development of the telescope.